Contributed by Daniel Puppe
Silicon is the second most common element in the Earth’s crust (after oxygen) and the seventh most abundant element in the universe. That means we can find silicon almost everywhere. Silicon plays a pivotal role in diverse living organisms comprising pro- and eukaryotes accumulating biogenic silicon in various siliceous structures (= biosilicification) – like idiosomic testate amoeba shells. In soils of terrestrial ecosystems we can find a lot of biogenic silicon forming different silicon pools. These pools can be separated into zoogenic, phytogenic, microbial and protistic ones (Fig. 1).
While scientific research has been focused especially on the phytogenic silicon pool (represented by so-called phytoliths), little is known about zoogenic, microbial and protistic silicon pools. The protistic silicon pool in soils comprises mainly terrestrial diatoms and idiosomic testate amoebae (some testates are shown in Fig. 2).
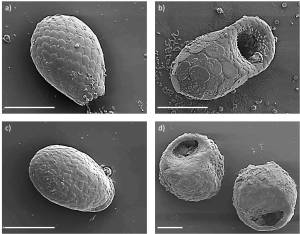
Fig. 2: Scanning electron microscope (SEM) micrographs of various idiosomic (a – c) and xenosomic (d) testate amoebae: a) Euglypha rotunda-like amoeba, b) Puytoracia bonneti (first record for Germany), c) Corythion dubium and d) two individuals of Centropyxis sphagnicola. Scale bars in all micrographs = 20 µm. Source: Puppe et al. 2014.
However, what is the relevance of biogenic silicon pools for silicon cycling? To understand this, we have to look at biogeochemical cycles at a global scale. Globally, silicon and carbon cycles are connected by weathering processes and fluxes of dissolved silicon from terrestrial to aquatic ecosystems (e.g. Sommer et al. 2006). When silicon is washed away into the oceans, it is used by marine diatoms for frustule synthesis (frustules are the siliceous cell walls of diatoms). Due to their worldwide distribution in very high abundances, diatoms are able to fix carbon dioxide (CO2) on a large scale (about 20 % of the photosynthesis on Earth is carried out by diatoms! See, e.g., Armbrust 2009). By consuming atmospheric CO2, diatoms thus have an effect on climate change, which is mainly caused by increasing atmospheric concentrations of the greenhouse gas CO2 since 1750 (IPCC 2013). The fluxes of dissolved silicon are affected by organisms that synthesize siliceous structures and consequently accumulate and recycle biogenic silicon in soils. In other words, the more silicon that is fixed in terrestrial ecosystems, the less silicon that arrives in the oceans, and as a consequence diatom production in the oceans decreases. Let´s visualize the main aspects of the processes described so far (Fig. 3).
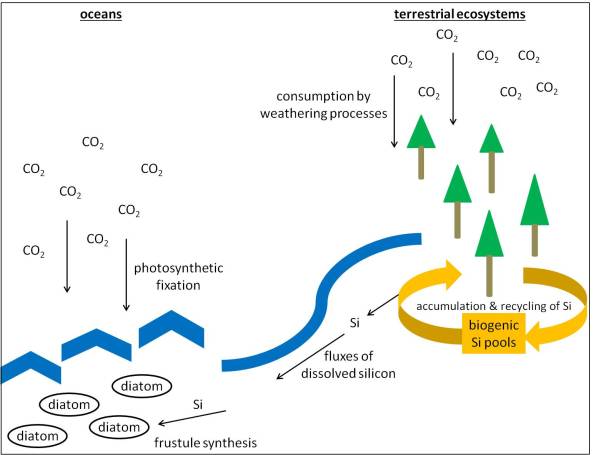
Fig. 3: Connections of global silicon (Si) and carbon (C) cycles and the influence of biogenic silicon pools (see descriptions in the text).
In two recent publications we analyzed protozoic silicon pools (represented by idiosomic testate amoebae) in initial (Puppe et al. 2014) and forested (Puppe et al. 2015) ecosystems. We found, that after (only!) 10 years of development idiosomic silicon pools in initial ecosystem states become comparable to the ones in forested ecosystems. In forest soils idiosomic silicon pools were relatively small (0.2 kg – 4.7 kg silicon per hectare in the upper 5 cm). Due to the fact that only intact shells were enumerated in our studies idiosomic silicon pools might be larger than calculated. However, there is no information on the quantity of this “platelet silicon pool”.
At the forested sites we further analyzed potential influences of abiotic factors (e.g. soil pH) and earthworms on idiosomic silicon pools. Surprisingly, no relationship between silicon supply (readily-available silicon in soils) and idiosomic silicon pools could be found, thus no silicon limitation for shell synthesis appeared in the field. Instead, idiosomic silicon pools showed a strong, negative relationship to earthworm biomasses. We concluded that earthworms control idiosomic silicon pools by direct (e.g. competition in the soil food web) and/or indirect mechanisms (e.g. change of habitat structure through burrowing activities). Earthworms themselves were strongly influenced by soil pH. Due to the fact that soil pH is a result of weathering and acidification, idiosomic silicon pools are indirectly, but ultimately controlled by soil forming factors, mainly parent material and climate. These results point to the potential relationships between soil fauna and the storage of biogenic silicon in terrestrial ecosystems. However, further research is needed to enlighten the complex biotic linkages in terrestrial biogeochemical silicon cycling and their importance for global silicon fluxes.
Annual biosilicification rates of living testate amoebae (17 kg – 80 kg silicon per hectare) in forested ecosystems were comparable to or even exceeded reported data of annual silicon uptake by trees. Just imagine it! Unicellular, microscopic organisms can out-compete multicellular, macroscopic ones in terms of annual silicon uptake. Given the worldwide distribution of testate amoebae, the importance of idiosomic silicon pools and corresponding biosilicification for (global) silicon cycling becomes clear.
Literature Cited
Armbrust, E.V. (2009). The life of diatoms in the world’s oceans. Nature, 459, 185-192.
IPCC, 2013: Climate Change 2013: The Physical Science Basis. Contribution of Working Group I to the Fifth Assessment Report of the Intergovernmental Panel on Climate Change. Stocker, T.F., D. Qin, G.-K. Plattner, M. Tignor, S.K. Allen, J. Boschung, A. Nauels, Y. Xia, V. Bex & P.M. Midgley (eds.). Cambridge University Press, Cambridge, United Kingdom and New York, NY, USA, 1535 pp.
Puppe, D., D. Kaczorek, M. Wanner & M. Sommer (2014). Dynamics and drivers of the protozoic Si pool along a 10-year chronosequence of initial ecosystem states. Ecological Engineering 70, 477-482.
Puppe, D., O. Ehrmann, D. Kaczorek, M. Wanner & M. Sommer (2015). The protozoic Si pool in temperate forest ecosystems – Quantification, abiotic controls and interactions with earthworms. Geoderma 243-244, 196-204.
Sommer, M., D. Kaczorek, Y. Kuzyakov & J. Breuer (2006). Silicon pools and fluxes in soils and landscapes – a review. Journal of Plant Nutrition and Soil Science 169, 310-329.